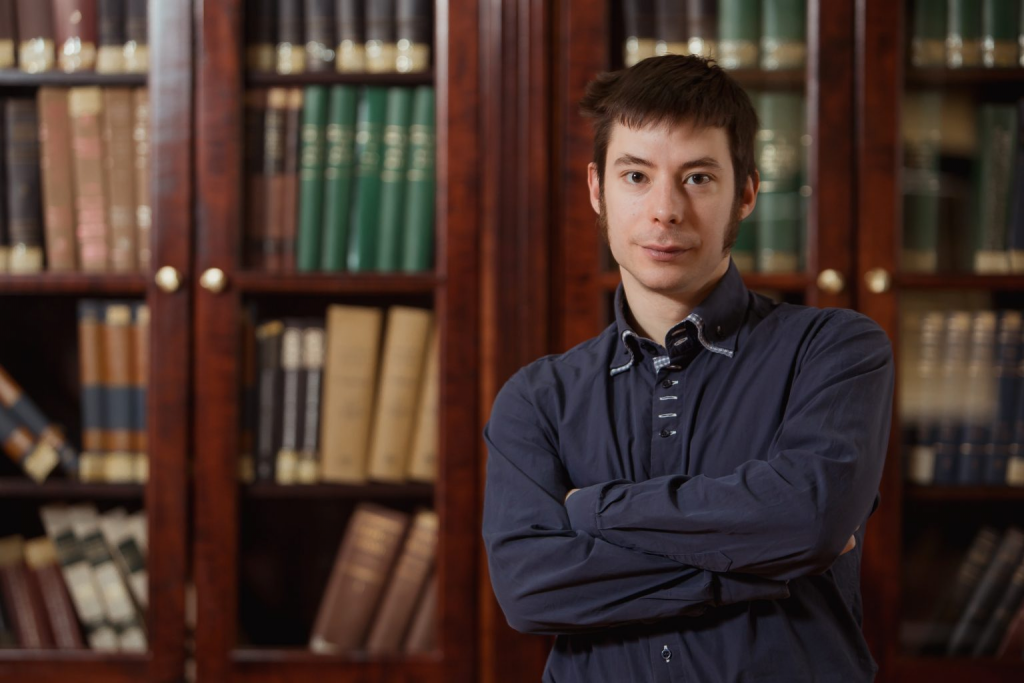
The scientific conference titled “Black Holes and Chaos” takes place from Wednesday, 4 September to Friday, 6 September at the prestigious Palace of the Serbian Academy of Sciences and Arts. The event attracted several distinguished researchers from renowned global institutions such as Berkeley, Harvard, Weizmann Institute of Science, Cambridge, and Santa Barbara.
For the last ten years, black holes have taken a central stage of both string theory and general quantum gravity, and the reason is due to two key factors: the black hole information paradox and they are an important stepping stone – since despite numerous complex problems (such as the above-mentioned information paradox), black holes are still simpler than many other systems in quantum gravity,’ says Dr Mihailo Čubrović, a researcher of the Institute of Physics Belgrade and one of the members of the Organizing Board of the Conference.
Much recognized in this field, Čubrović has published papers in such a journal as the Journal of High Energy Physics, Physical Review и Science, and at the Institute of Physics, he is one of the returnees who started their career abroad before returning to Serbia. He received his PhD from the University of Leiden and continued his work at the University of Cologne and then came back to the Institute of Physics Belgrade in 2017. Along with Marija Tomašević from Amsterdam, Kiril Hristov from Sofia, Sašo Grozdanov from Ljubljana, and Branislav Cvetković and Ivan Đikić from the Institute of Physics Belgrade, he organized the conference on black holes and chaos.
Belgrade hosts scientific conferences daily, however, this one is particularly interesting because it focuses on cosmological objects that are at the forefront of modern science while attracting some of the most renowned experts in the field. The goal of this event is to connect young researchers from our regions with leading global researchers in the field related to black hole studies. The conference is jointly organized by the Institute of Physics Belgrade and the Mathematical Institute of SASA.
‘Now, details of black hole physics have become accessible through observational techniques, which makes the subject even more fascinating’, states Čubrović in an interview for the Institute of Physics’s internet portal, conducted by Jovana Nikolić and Slobodan Bubnjević from the Communication Department of the Institute.
How much can be discovered about black holes through experimental techniques and available instruments at such a developmental level compared to the theoretical predictions?
Mihailo Čubrović: The fundamental challenge in observing black holes is clear – since they are black and do not emit light, it is not possible to observe them directly. However, there are roundabout ways. One such method is through gravity pull – if a black hole is part of a binary or multi-star system, the mass of a black hole can be established by other stars’ paths. Similarly, rough estimates of black holes’ masses in the centres of galaxies, including our own, can be made, however, one has to bear in mind that we are talking about a humongous number of stars orbiting the black holes at the centre and their orbits are rather complicated and chaotic due to numerous flashes and passings, therefore determining the mass of these black holes is more statistical in nature.
Nevertheless, determining mass as the only data is not an impressive achievement. The second indirect way, now long known, we owe to the effect of gravity lense – it enables us to learn more. Since gravity affects light as well, which is the reason why black holes are black, the black hole deflects the light passing at a certain distance from it – not enough to absorb it, but enough to deform the source image (for example, some star, further away from the black hole, but which can be seen in almost the same direction).
Based on this deformation, we can draw conclusions about the space-time metric close to the black hole, which in turn provides us with information about whether the black hole is rotating, the diameter of its event horizon, and a few other characteristics. This method offers a better understanding, but it relies on chance – specifically, whether there is a light source behind the black hole whose light is deflected by the black hole’s gravitational field.
The third approach involves observing the accretion disk. While the previous method observed how the black hole’s gravity affects light, in this one, we focus on how its gravity affects matter – for example, gas or plasma of a nearby star (once again in binary or multi-star system) which the black hole pulls in. Before collapsing into the black hole, this matter orbits it for some time, and based on the shape and brightness of the accretion disc we can make conclusions about these orbits, hence we get information about the mass, size and rotation speed of the black hole.
The first images of black holes garnered a lot of attention seven years ago
Through the application of these methods, the existence of astrophysical black holes has definitely been confirmed. As we said before, they can either originate from former stars with a mass large enough to collapse into a black hole after a supernova explosion, and we detect them then if they are members of multi-star systems, or they can be supermassive black holes located at the centres of galaxies, likely formed by collisions and mergers of countless ordinary stars, due to their dense concentration in the centre of the galaxy. However, truly interesting things in the field of observation started happening in 2017. It was then that the Event Horizon Telescope (EHT), a system of several large radio telescopes (operating mostly in the millimetre and submillimetre range) on different continents, set up to work as a single interferometer, captured the shadow and photon ring of the black hole at the centre of the M87 galaxy. Following this, the black hole in the centre of our galaxy was also recorded.
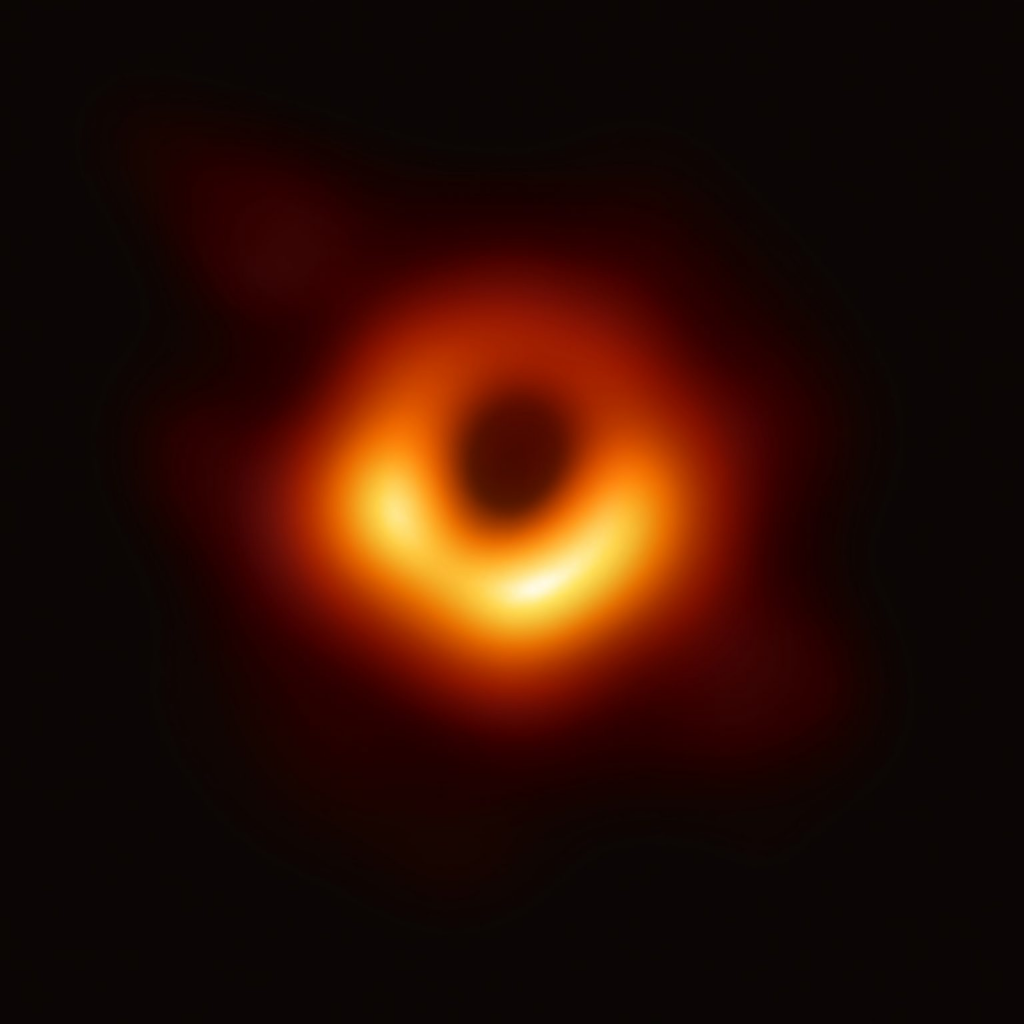
How is it at all possible to do this?
First, we have to explain what the shadow and photon ring represent. The black hole has a horizon – this is its boundary; whatever crosses the horizon does not return. Just outside the horizon, there are orbits approaching it – they haven’t collapsed yet, but most will; for even a small disturbance is enough to make this happen. Further from the horizon, quite the contrary, most orbits are safe and only the rare ones on specific trajectories will end up in the black hole. These two regions are separated by a photon sphere – an area that consists of orbits that are exactly at the saddle points. These orbits circle the photon sphere indefinitely, with some of them being stable and some unstable. Inside the photonic sphere, there is the shadow of the black hole – this is the part that appears dark despite not belonging to the black hole; the light can escape it but most rays gradually get sucked into the black hole by means of small disturbances and this is why the shadow seems dark.
It turns out that a lot of information can be drawn from the orbits in the photon sphere, from their structure and the shape of the black hole’s shadow – far more than with the previously mentioned methods. Interferometric observations such as the ones made with the EHT provide a relatively fine structure of the photon sphere. For instance, the thermodynamic properties of the black hole, such as the temperature, as well as the spectrum of quasi-normal modes can be determined from it. Quasi-normal modes are damped oscillations in spacetime that occur when a black hole responds to some disturbance, for example, absorption of light or matter. The spectrum of quasi-normal modes is something like the DNA of a black hole – even the effects of quantum gravity can partially be reconstructed from it, which is perhaps the most exciting thing of all. However, we should not rejoice prematurely – the precision of EHT images is still limited, so the spectrum of quasi-normal modes has only been very roughly approximated. Nevertheless, the first step has been taken, and how much we can derive from the limited observational data is also a matter of the advancement of the theory itself.
How much do we learn from this ‘observational’ data about the state of the black hole?
This raises a crucial question for theorists: can the microstate of a black hole be approximated from a limited amount of data? Well, it turns out that some, with the accent on some, properties can indeed be determined from a minimal amount of data thanks to the universal character of these holes. The simplicity of black holes mentioned at the beginning becomes evident here. There are several both important and simple relations that black holes condone. For instance, black holes are thought to be the most chaotic objects in nature, as suggested by the MSS (Maldacena-Shenker-Stanford) Hypothesis.
Chaos unexpectedly became a topic closely related to black holes.
The instability exponent (Lyapunov exponent) of black holes is directly linked to their temperature and can be derived from the quasi-normal modes—specifically, from the imaginary part of those modes. In thermodynamic terms, black holes are described by the standard laws of thermodynamics (from the zeroth to the third law), and quantities such as mass, angular momentum, and electric charge can already be measured through observations. However, this is where it gets trickier because of the information paradox. But, that is a vast topic beyond the scope of this discussion, and let’s just say that, when we delve into and consider Hawking radiation, i.e. black hole radiation (which, though impossible according to classical physics, must exist under quantum mechanics because every object radiates at a finite temperature), this radiation must behave differently from the usual thermal radiation (for example, the radiation of a light bulb filament). Otherwise we either have to give up quantum mechanics or the quasi-classical picture of gravity. It seems more reasonable to abandon the traditional view of gravity (after all, we know that it must be adjusted to explain the deep quantum regime phenomena).
Can we avoid the information paradox?
An important breakthrough in this direction came in 2019 with the introduction of the idea of replicas and wormholes. In a sense, the geometry of a black hole is not a unique one, we should consider all possible geometries complying with the boundary conditions imposed by the theory, including wormholes which connect different spaces. When we calculate the sum of all replicas, the information paradox probably could be sidestepped. However, what are the observable consequences of such a scenario? It appears that they should be sought in small but finite deviations from the simple and universal picture we usually have of black holes. For instance, the Lyapunov exponent of a black hole could be close to the maximum value (given by the MSS inequality), but still slightly smaller than it; the thermodynamics would be close to the usual equilibrium thermodynamics, but still with a small deviation from equilibrium. Some of these hypotheses will be testable through observations in the near future.
Can we now claim a new understanding of these enigmatic objects?
We can conclude that while the connection between theory and experiment—primarily through observations—remains limited, they are drawing closer. The prospect of directly observing some quantum effects is particularly appealing, and that seemed far-fetched for a long time. We repeatedly talked about instability and chaos in our discussion: from the Lyapunov exponents of black holes to the stable and unstable orbits on the photon sphere, and the non-equilibrium thermodynamics. The chaos theory, black holes, and string theory are closely related nowadays, with many shared problems. Indeed, a decade ago, such developments would have seemed almost unbelievable! This is also the driving force behind organizing such a conference, focused specifically on diagnosing black holes through chaos and, in turn, understanding chaos through black holes. The timing seems ideal to intersect these fields, paving the way for further advancements toward quantum gravity.
Photos: Bojan Džodan, EHT